My research is focused on determining how nervous systems encode and retain information, and how alterations in neuron function lead to behavioural changes in an organism. Specifically, I am interested in how we can manipulate the nervous system to enhance learning and memory processes. I specialize in using multilevel analyses to draw strong connections between behaviour, neuron function, and anatomical or cellular changes. My research has evolved into studying the following themes:
Learning and Memory Suppressors.
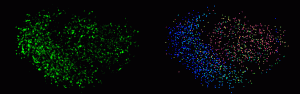
3D projections of synaptic GFP and their particle centroids
Research over the last ~5 decades has focused intensely on revealing those molecular and cellular functions that are required for memory formation. Interestingly however, it has become increasingly clear that the brain is designed with processes that also constrain memory formation, such that reducing the activity of these molecules enhances behavioral memory. Genes encoding these products are termed ‘memory suppressor genes’, thus the normal function of these molecules limit some learning and memory process. Arguably, the knowledge of the negative regulators of memory is equally important to that of positive ones. Moreover, memory suppressors are highly relevant for understanding enhanced and compromised memory that occurs in the human population. Savant syndrome, for example, is characterized by remarkable memory capacity, often occurring in individuals with autism spectrum disorder (ASD), while post-traumatic stress disorder (PTSD) presents as maladaptive, abnormally strong memories, perhaps arising from dysregulation of memory suppressor genes and/or their products. I have identified several new memory suppressor genes, and am currently working to reveal how they function. The existence of memory suppressors naturally leads to the question: Why is there a biological need to limit memory storage and retention in the nervous system? This question has yet to be explored, but some hypotheses are that they allow for behavioural flexibility in ever-changing environments, they help to promote the formation of accurate memories (filtering out probabilistically unlikely associations), or perhaps they have a separate role in promoting normal social interactions. This is a question that I plan to pursue in the future.
Social Isolation and the Brain.
Loneliness increases the risks for both mental and physical health problems. Not only are loneliness and social isolation related to higher incidences of anxiety, depression, dementia and other mental health problems, they also increase rates of cardiovascular disease and all-cause mortality. Despite the generally accepted assertion that loneliness and social isolation result in a variety of behavioural changes, surprisingly little is known about the neuroanatomical changes or cellular mechanisms that lead to these changes. Inspired by events of the COVID-19 pandemic, I have developed a social isolation model in Drosophila to study this question, demonstrating that learning, sleep, locomotion, and social interactions are altered in socially isolated flies, similar to humans and mice. My lab now aims to identify specific neurons and proteins responsible for these changes using this model system to reveal the mechanisms through which social isolation lead to synaptic alterations in the brain.
Synaptic Vesicle Biogenesis.
Synaptic vesicles (SVs) package neurotransmitters and are the fundamental unit for communication across the synapse of all multicellular lifeforms with a nervous system. The release of neurotransmitters via a highly regulated and orchestrated fusion of SVs to the plasma membrane of a cell is indispensable for the physiological and behavioural functions necessary for multicellular life. Consequently, mutations that affect SV function are associated with a large range of disorders, including epilepsy, locomotor, neurodevelopmental and neurodegenerative disorders. Thus, unsurprisingly there has been a concerted effort placed into deciphering the cellular machinery supporting SV function at the synapse. Curiously, comparatively little attention has been directed to examining the regulation of their de novo biogenesis at the cell body or the cellular controls dictating the numbers of SVs at the synapse. This sets the synaptic strength of the neural network, altering behaviour of the organism. The lack of understanding in this area of SV biology is likely due to the difficulty in experimentally approaching this question because of confounding alterations in neuron or synapse morphology. My prior work investigating the mechanisms of a novel memory suppressor has unexpectedly revealed an experimental entrée into identifying molecules that regulate the numbers of SVs a neuron creates and stores in their presynaptic compartments. Thus, my lab is currently pursuing this line of inquiry to identify the molecules that set this parameter and to identify how they function.